Advances in subsea fiber cable technology
Before subsea cables were laid across the world’s oceans, information traveled by written letters, carried on a ship. By the mid-19th century, copper cables had successfully connected Europe to North America, allowing telegraph messages to relay news in minutes instead of weeks. This had a profound economic effect on government, business, and individual lives. By 1890, telegraph cables had long been constructed across oceans and continents, and transmission speeds had evolved greatly compared to earlier systems. However, reaching speeds approaching just 100 words per minute would still take a long time.
Long ago we adapted much of that early ocean engineering to laying cables with optical fibers at the core, and we have witnessed generations of electronic equipment that uses light to put information on that fiber, vastly increasing capacity. At the same time, society’s demand for information and connectivity has increased by orders of magnitude, resulting in powerful computing systems and data centers spread across the world.
Modern subsea cable systems can deliver over 200 Tbps of lit capacity and span tens of thousands of kilometers. The ability to reach these distances with such enormous capacity has only been achievable through many innovations over a long time.
Capacity, fibers, and modulation
Those early subsea cables needed high-voltage transmitters designed to overcome their inherent high resistance. At the time, the cable’s inductive and capacitive reactance was not well understood and methods to cope with it took years to develop. The result was that these early systems limped along at a capacity of 10-15 words per minute. These systems were gradually improved, eventually supporting voice transmission around 1945 on short spans. The first transatlantic cable to carry voice service, TAT-1, was put in service in 1965, 100 years after the first successful telegraph cable (Figure 1).
Subsea cable evolution sped up rapidly in the next decades, with the introduction of armored optical cable in the early 1980s and commercial deployment in TAT-8, the first optical transatlantic cable, placed in operation in 1988. Many design challenges had to be overcome, including physical durability, optical loss, spectral dispersion, and methods to amplify optical signals mid-span. The development of EDFAs, low-loss single-mode fiber, and other innovations made TAT-8 practical. It was in operation for 14 years delivering service at 280 Mbps. Further developments including multiple fiber cores and the use of WDM scaled cable capacity greatly. By the time TAT-14 was placed in service in 2001, it offered a lit capacity of 3.2 Tbps, over four orders of magnitude greater than TAT-8’s capacity in just 12 years.
As with other optical span types, the methods used to put information on fiber also evolved greatly. Intensity modulation of DFB lasers with direct detection (IM-DD) worked well enough at first, and capacity additions were realized through the addition of wavelengths in a WDM system. External modulation added capacity and reach by overcoming the shortcomings of direct modulation. But the biggest breakthrough in the tradeoff of system capacity versus span reach came through the introduction of coherent optical detection systems around 2008.
Coherent detection opened the door to advanced modulation techniques that make use of the optical carrier’s phase and amplitude to encode data. That means more bits get encoded in each symbol and spectral efficiency is greatly increased. It also enables use of digital processing techniques to maximize link performance. These advances have colloquially come to be called coherent optical engines – components that optimize system performance to a point very close to the Shannon limit.
Unique subsea cable challenges
Subsea transmission presents unique challenges both in cable construction and operation. Much as in early copper systems, overcoming physical impairments presented by thousands of kilometers of cable is crucial to the design, installation, and operation of a subsea optical cable.
While optical fibers have improved greatly in their loss and dispersion characteristics, amplification is still needed to overcome cable losses. These repeater systems need to be powered remotely, from the cable landing station to locations roughly every 100 km. As an example, the SEA-ME-WE-5 cable system is among the longest in the world, connecting Singapore to Europe, with multiple branch points along its length of 20,000 km and offering a lit capacity of 17 Tbps. Completed in 2016 by Alcatel Submarine Networks, this system required clever engineering of cable spans, repeaters, and submarine line termination equipment (SLTE).
Modern Technologies
Several technological advances provide enormous benefits to subsea cable operators in meeting their needs for capacity, manageability, reliability, and economy. These include the use of coherent detection and fine-tuning through error correction, modulation shaping, baud rate optimization, and other technologies (Figure 2).
Coherent Detection
Coherent detection enables more information to be encoded in each optical wavelength. Multi-level quadrature phase-shift keying (QPSK) and quadrature amplitude modulation (QAM) became economically feasible when digital signal processing (DSP) engines reached a favorable performance and cost point. Around 2010, 32-Gbaud DSPs were used to build links using QPSK modulation, yielding 8-Tbps fiber capacity in the optical C-Band. Advances continued through the next decade, allowing operation at 64-67 Gbaud with 64QAM modulation and, more recently, 90- to 96-Gbaud symbol rates using 7-nm process geometry DSPs, operating at up to 800 Gbps per wavelength. This trend of smaller DSP process technology increased baud rate; the resultant increased system capacity is expected to continue through a few generations though physical limits are increasingly challenging. Coherent DSPs at 5 nm are close to commercial availability and wavelengths exceeding 1 Tbps are expected soon.
Fine Tuning
The evolution of DSPs does more for optical transmission than support of coherent detection. It also provides a computational capability that can be used in several ways, including forward error correction (FEC), probabilistic constellation shaping (PCS), and continuous bit-rate adjustment. These mechanisms help optimize optical link performance, both for terrestrial and subsea applications. Indeed, for a given optical fiber channel, maximum spectral efficiency can be obtained by matching FEC, modulation format, and symbol rate to the underlying channel conditions.
FEC is a means to reduce the effects of transmission errors in a noisy channel by predicting when an error has occurred and correcting it. This is done using redundant data sets that can be interpreted through an algorithm and predictively decide when an error has occurred. This additional data is called the “FEC overhead,” which yields a coding gain applied to a signal-to-noise ratio that results in a specified bit error rate (BER).
The concept is quite old; the Reed-Solomon code was developed in the early 1960s. FEC has evolved through the use of different coding schemes, including concatenated FEC, low-density parity check, and, most recently, the addition of soft-decision FEC (SD-FEC) where error probabilities and more advanced computation enable us to more accurately predict and correct errors while using less overhead capacity. Together, modern SD-FEC can provide net coding gain at nearly 12 dB with just 16% of overhead.
Another optimization is adjusting the modulation constellation using probabilistic methods: probabilistic constellation shaping (PCS). The idea is to adjust energy expended at points in a particular modulation constellation to reflect the noise characteristics of a particular channel more accurately. By focusing energy on lower amplitude symbols as the data rate decreases, PCS transforms a square constellation into a more Gaussian-like shape (Figure 3). For example, we could think of the actual modulation format as neither 16QAM nor QPSK but rather somewhere in-between, with constellation points towards the center more frequently excited. The result is link performance very close to the Shannon limit at a particular symbol rate and optical span.
Related to PCS is the use of very fine adjustments in link baud rate to optimize spectral efficiency, line capacity, and optical span reach. For a fixed bit rate, shaped modulation format, and FEC scheme, we can adjust the baud rate (bit rate per symbol on the modulation constellation) and realize a tradeoff in link capacity versus span length. As baud rate increases, span reach increases incrementally while link capacity decreases proportionally. For practical applications in either terrestrial or subsea systems, baud-rate selection can result in a non-optimal operating point, where system reach or capacity is not as good as it could be. By adjusting the baud rate in fine increments, roughly around 0.1 Gbaud, system flexibility increases to operate at a desirable span length for a given capacity, and within a desired optical channel plan.
In subsea cable systems, such adjustment enables the operator to increase the number of deployed channels (and the resulting total lit capacity), increase system link margins, or reduce the number of operating optical transponders — all through fine baud rate adjustment. Together, these adjustments in baud rate allow the operator to optimally fill the subsea cable’s optical spectrum. This “water filling” technique yields economic benefit in fully utilizing a subsea cable at maximum capacity and spectral efficiency.
What lies ahead?
Coming generations of DSPs are sure to continue improvements and optimizations in link performance as described above. These devices will push us closer to operation near the Shannon limit while also offering better SLTE economies. But other subsea networking innovations will also be important, including subsea cable branching and advances in physical fiber construction.
We already can deploy subsea branching units that allow for remote wavelength routing to different landing sites. This capability is useful in building protection switching, traffic diversity planning, or dynamic traffic capacity routing. We should expect the utilization of these capabilities to increase as various end-users seek ever-increasing amounts of capacity to different and changing locations across the globe.
Finally, innovations in the fiber cable itself look promising. Spatial division multiple (SDM) provides the ability to scale capacity through the addition of new fiber cores to each cable. In the near term, SDM will put more fiber pairs, using narrower fiber coatings, in a single cable. In the longer-term, multiple fiber cores will be constructed in a single coated fiber, offering an 8-10X increase in capacity per fiber.
Together, all these innovations are helping satisfy the need for increased global connectivity, Today, much as in 1890, most of the globe is connected through subsea cables. Capacity and performance will continue to improve and have a vast impact on global commerce.
Chris Janson is senior product marketing manager of optical networking at Nokia. In this role, he follows trends in optical networking technology and their application to finance, healthcare, utilities, government, and educational customers.
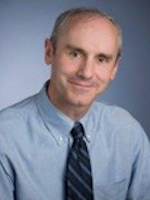
Chris Janson | Product Marketing Manager, Optical Networking
In his current role with Nokia, Chris Janson follows trends in optical networking technology and their application to finance, healthcare, utilities, government and educational customers. Mr. Janson has been a speaker at many conferences including Interop 2014, Internet2 annual technology meetings, and a 2015 series of executive forums sponsored by CenturyLink and CIO magazine. He has also shared his work through many webinars, written publications, on-line videos and articles. Mr. Janson also serves on the boards of directors of the Rural Telecommunications Congress and the non-profit OpenCape Corporation. He holds an MBA from Boston University and Bachelor of Science in engineering from Wentworth Institute of Technology.